
Diary of a Supernova: How (Some) Stars Blow Up
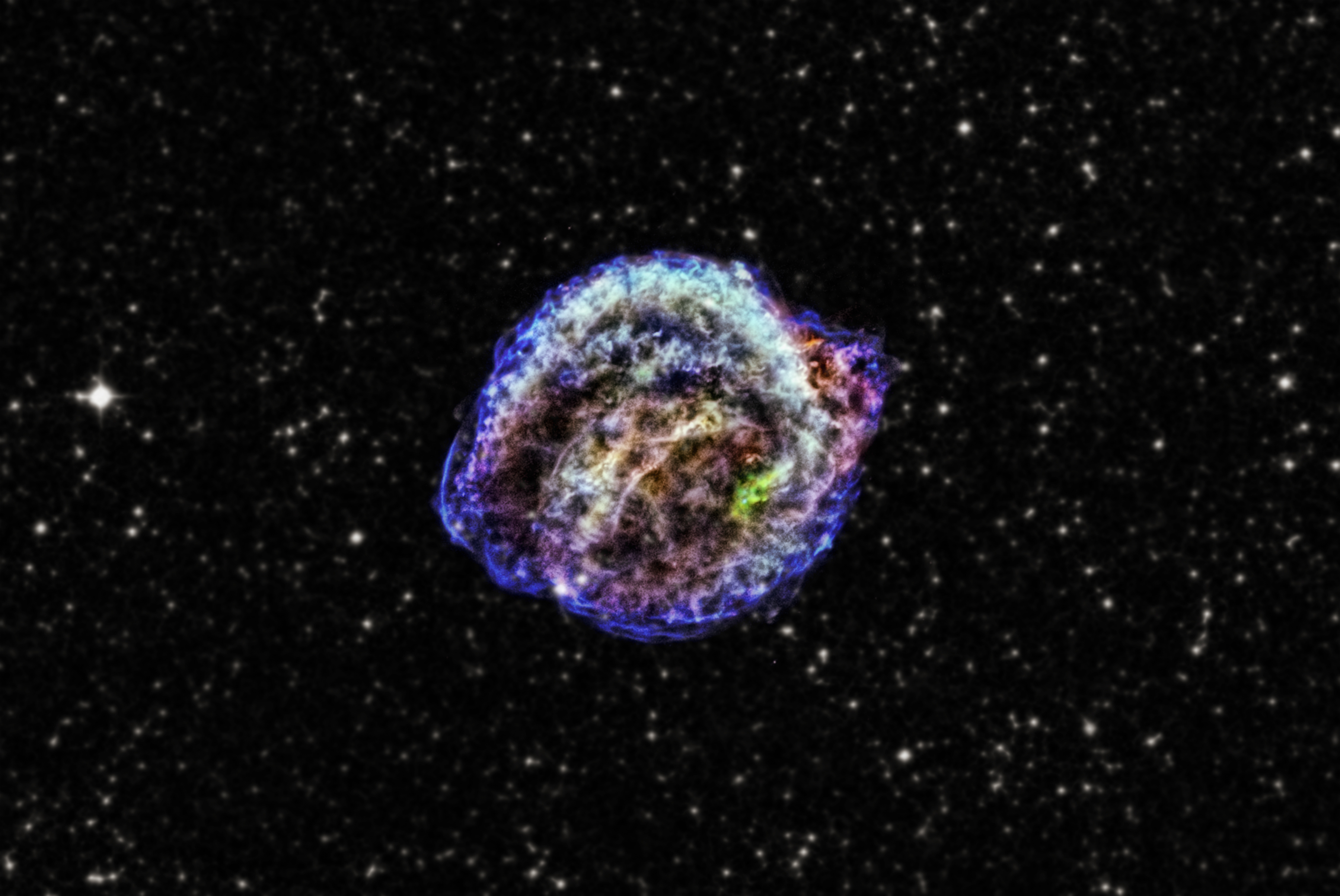
Paul Sutter is an astrophysicist at The Ohio State University and the chief scientist at COSI science center. Sutter is also host of Ask a Spaceman and Space Radio, and leads AstroTours around the world. Sutter contributed this article to Space.com's Expert Voices: Op-Ed & Insights.
Everything in the universe someday comes to an end. Even stars. Though some might last for trillions of years, steadily sipping away at their hydrogen reserves and converting them to helium, they eventually run out of fuel. And when they do, the results can be pretty spectacular.
Our own sun will make a mess of the solar system when it enters the last stages of its life in 4 billion years or so. It will swell, turn red (consuming Earth in the process) and cast off its outer layers, giving one last gasp as a planetary nebula before it settles down into post-fusion retirement as a white dwarf. [Supernova Photos: Great Images of Star Explosions]
The most spectacular deaths, though, are reserved for the most massive stars. Once an object builds up to at least eight times the mass of the sun, interesting games can be played inside the core, with … explosive results.
Walking the nuclear line
Any star, no matter how massive, walks a thin tightrope. On one side is the crushing gravity of the star's own weight, which provides the pressures and temperatures necessary to achieve nuclear fusion in the core and turn hydrogen into helium. But that fusion process releases energy, which puts the star in a more expansive mood than gravity does alone.
To understand how this works, let’s work through a thought experiment. Imagine that the gravity were to increase a tiny bit, then the increased pressure would raise the intensity level of the fusion reactions, which, in turn, would release more energy and thus prevent further collapse of the star. And on the opposite end, if the fusion party were to get just a little bit wilder, it would cause to star to overinflate, lessening the grip of gravity and easing the pressure in the core, cooling things off.
This balancing act enables a star to last millions, billions and even trillions of years.
Get the Space.com Newsletter
Breaking space news, the latest updates on rocket launches, skywatching events and more!
Until it doesn't.
The game can be played as long as there's fuel to keep the lights on. As long as there's a sufficient supply of hydrogen near the core, the star can keep cranking out the helium and keep resisting the inevitable crush of gravity.
A crushing force
I'm not just using a flair of language when I describe the crush of gravity as inevitable. Gravity never stops, never sleeps, never halts. It can be resisted for a long time, but not forever.
As a star ages, it builds up a core of inert helium. Once the hydrogen supply exhausts itself, there's nothing to stop the infalling weight of the surrounding material. That is, until the core reaches a scorching temperature of 100 million kelvins (180 million degrees Fahrenheit), at which point helium itself begins to fuse.
Hooray, the party's back on! Well, for a while, at least. Helium fusion isn't as efficient as good ol' hydrogen, so the reactions happen at an even faster pace to compete with gravity.
While the "main sequence" of a star's life may last hundreds of millions of years as it happily burns hydrogen, the helium phase barely lasts a single million.
The product of helium fusion is carbon and oxygen, and the same game gets played again, but at even higher temperatures and shorter timescales. Once the helium is sucked dry, the core collapses and intensifies to 1 billion K (1.8 billion degrees F), allowing those new elements to get their turn.
Out of control
Then, silicon fuses at around 3 billion K (5.4 billion degrees F) in the core, generating iron. Surrounded by plasmatic onion-like layers of oxygen, neon, carbon, helium and hydrogen, the situation at the center starts to get dicey.
The problem is that, due to its internal nuclear configuration, fusing iron consumes energy rather than releases it. Gravity keeps pressing in, shoving iron atoms together, but there's no longer anything to oppose its push.
In less than a day, after millions of years of peaceful nuclear regime changes, the star forms a solid core of iron, and everything goes haywire.
In a matter of minutes, the intense gravitational pressure slams electrons into the iron nuclei, transforming protons into neutrons. The small, dense neutron core finally has the courage to resist gravity, not by releasing energy but through an effect called degeneracy pressure. You can only pack so many neutrons into a box; eventually, they won’t squeeze any tighter without overwhelming force, and in the first stages of a supernova explosion, even gravity can't muster enough pull.
So now you have, say, a couple dozen suns' worth of material collapsing inward onto an implacable core. Collapse. Bounce. Boom.
The inside-out inferno
Except there's a stall. The shock front, ready to blast out from the core and shred the star to stellar pieces, loses energy and slows down. There's a bounce but no boom.
To be perfectly honest, we're not exactly sure what happens next. Our earliest simulations of this process failed to make stars actually blow up. Since they do blow up in reality, we know we're missing something.
For a while, astrophysicists assumed neutrinos might come to the rescue. These ghostly particles hardly ever interact with normal matter, but they're manufactured in such ridiculously quantities during the "bounce" phase that they can reinvigorate the shock front, filling its sails so it can finish the job.
But more sophisticated simulations in the past decade have revealed that not even neutrinos can do the trick. There's plenty of energy to power a supernova blast, but it's not in the right place at the right time.
The initial moments of a supernova are a very difficult time to understand, with plasma physics, nuclear reactions, radiation, neutrinos, radiation — a whole textbook's worth of processes happening all at once. Only further observations and better simulations can fully unlock the final moments of a star's life. Until then, we can only sit back and enjoy the show.
Learn more by listening to the episode "Why do some stars blow up?" on the Ask A Spaceman podcast, available on iTunes and on the web at http://www.askaspaceman.com. Thanks to @ionut_branea and @ThomasPadden1 for the questions that led to this piece! Ask your own question on Twitter using #AskASpaceman or by following Paul @PaulMattSutter and facebook.com/PaulMattSutter. Follow us @Spacedotcom, Facebook and Google+. Original article on Space.com.
Join our Space Forums to keep talking space on the latest missions, night sky and more! And if you have a news tip, correction or comment, let us know at: community@space.com.
Paul M. Sutter is an astrophysicist at SUNY Stony Brook and the Flatiron Institute in New York City. Paul received his PhD in Physics from the University of Illinois at Urbana-Champaign in 2011, and spent three years at the Paris Institute of Astrophysics, followed by a research fellowship in Trieste, Italy, His research focuses on many diverse topics, from the emptiest regions of the universe to the earliest moments of the Big Bang to the hunt for the first stars. As an "Agent to the Stars," Paul has passionately engaged the public in science outreach for several years. He is the host of the popular "Ask a Spaceman!" podcast, author of "Your Place in the Universe" and "How to Die in Space" and he frequently appears on TV — including on The Weather Channel, for which he serves as Official Space Specialist.