The 1st Milky Way black hole image was groundbreaking — the next could be even better
The Event Horizon Telescope’s new abilities will help it see more details in the ring of light surrounding a black hole’s shadow.
The Event Horizon Telescope (EHT), which is a collaboration of radio telescopes all around the world that operate in unison to image supermassive black holes, has achieved its finest resolution yet. In the future, this accomplishment could lead to images of the ring of light around a black hole's event horizon that are 50% sharper, resolving hitherto unseen details and producing movies of how the black holes change as they spin.
The EHT works on the principle of "very long baseline interferometry," or VLBI for short. This involves tapping into a network of telescopes across continents that all work collectively to observe the same object, combining their data in the process. The wider the distance between the two farthest telescopes in the network, the greater the resolution, and the more telescopes there are in the network, the greater the sensitivity.
The EHT managed to image the black hole in the center of our Milky Way galaxy, Sagittarius A*, as well as the black hole in the center of the elliptical galaxy M87, M87* — marking the first two black hole images captured by humanity — because it has a huge baseline. Think of the baseline as being the telescope's aperture. The EHT's most southern telescope is the South Pole Telescope, while its most northern station is the Greenland Telescope, which means the network spans almost top to bottom of the planet.
Along with the baseline factor, wavelength has a role to play, with lower wavelengths achieving higher resolution. The historic images of the black hole at the center of our galaxy and M87 were captured at a radio wavelength of 1.3mm. At this wavelength, the "photon ring," which is the torus of emission around the event horizon with the black hole's dark shadow inside it, appears blurred — particularly in the case of Sagittarius A*. This is because the radio emission coming from the black hole is being partially scattered by ionized gas in the interstellar medium between us and the object itself. This results in the light becoming smeared across an angular scale, comparable to the resolution of the EHT at 1.3mm. The smearing effect would be drastically less apparent at shorter wavelengths.
Related: Stunning Photos of Our Milky Way Galaxy (Gallery)
To this end, for the first time ever, the EHT has been able to conduct VLBI at a shorter wavelength of 0.87mm.
"With the EHT, we saw the first images of black holes by detecting radio waves at the 1.3mm wavelength, but the bright ring we saw, formed by light bending in the black hole's gravity, still looked blurry because we were at the absolute limits of how sharp we could make the images," said Alexander Raymond, of NASA's Jet Propulsion Laboratory, in a statement. "At 0.87mm, our images will be sharper and more detailed, which in turn will likely reveal new properties, both those that were previously predicted and maybe some that weren't."
Get the Space.com Newsletter
Breaking space news, the latest updates on rocket launches, skywatching events and more!
Achieving VLBI at 0.87mm is not an easy thing, hence why it hasn't been accomplished before. One of the difficulties has to do with water vapor in the atmosphere tending to absorb radio waves at this short wavelength, so weather would have to be very dry at all the EHT's observing sites.
As for those observing sites, this particular VLBI experiment involved both the Atacama Large Millimeter/submillimeter Array (ALMA) and the Atacama Pathfinder Experiment in Chile, the Institute for Radio Astronomy in the Millimeter Range (IRAM) 30-meter telescope in Spain, the Northern Extended Millimeter Array (NOEMA) in France, the Submillimeter Array (SMA) on Mauna Kea in Hawaii and the Greenland Telescope, all of which are stations for the EHT. Probing numerous quasars at 0.87mm, they achieved a resolution of 19 microarcseconds.
How small is 19 microarcseconds? Well, the sky is divided into 360 degrees, with each degree consisting of 60 arcminutes, and each arcminute is further subdivided into 60 arcseconds. A microarcsecond is a millionth of an arcsecond, so 19 microarcseconds is like being able to resolve a bottle-top on the surface of the moon. It's the greatest resolution for an astronomical image ever achieved solely from the surface of the Earth (although combinations of ground- and space-based telescopes have achieved similar resolutions in the past). Raymond and his colleagues actually think the EHT could resolve down to just 13 arcseconds when operating at full capacity (for example, with the inclusion of the South Pole Telescope, which was not involved in the 0.87mm test).
The intention now is to apply this breakthrough while taking new images of Sagittarius A* and the supermassive black hole in M87.
"The time is right, as the new detections prove, to advance to 0.87mm," said Remo Tilanus of the University of Arizona, who is the EHT's Operations Manager.
The greater resolution won't just sharpen the image of the photon ring around each black hole, but also more precisely depict their shape and size, allowing more accurate estimates of the black holes' spin rates and angle to us. It will also bring into range supermassive black holes in other galaxies, and allow us to see closer to the base of relativistic jets shooting out from active black holes such as in quasars. This could provide more answers into how a black hole's magnetic field produces jets that move at almost the speed of light and extend out into deep space for thousands of light-years.
In addition to now being able to operate at a shorter wavelength, there are plans in place for major modifications to the EHT, in a program called "next generation EHT," or "ngEHT" for short. This will add new telescopes to the existing EHT infrastructure at locations around the world optimized to provide the greatest baselines and sensitivity, as well as improve detector facilities at the existing members of the collaboration so they can observe black holes at multiple wavelengths between 3mm and 0.87mm simultaneously.
All in all, the next generation EHT is expected to increase the sharpness and clarity of black hole images by a factor of 10, perhaps even enabling high-resolution movies showing changes in the photon ring around a black hole's event horizon over time as the black hole spins and accretes more matter from the surrounding space.
"These VLBI signal detections at 0.87mm are groundbreaking since they open a new observing window for the study of supermassive black holes," said Thomas Krichbaum of the Max Planck Institute for Radio Astronomy in Germany in a statement issued by the European Southern Observatory.
The results of this groundbreaking VLBI experiment were published on Aug. 27 in The Astronomical Journal.
Join our Space Forums to keep talking space on the latest missions, night sky and more! And if you have a news tip, correction or comment, let us know at: community@space.com.
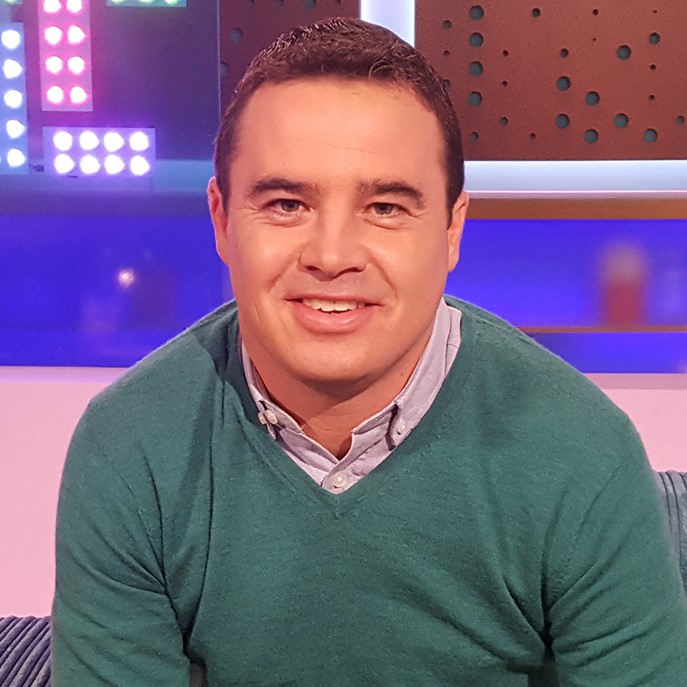
Keith Cooper is a freelance science journalist and editor in the United Kingdom, and has a degree in physics and astrophysics from the University of Manchester. He's the author of "The Contact Paradox: Challenging Our Assumptions in the Search for Extraterrestrial Intelligence" (Bloomsbury Sigma, 2020) and has written articles on astronomy, space, physics and astrobiology for a multitude of magazines and websites.
-
Questioner "...light bending in the black hole's gravity,..."Reply
Light follows the geometry of space-time exclusively.
The gravitational effect can not be involved because light/EM has no resting mass to favor/linger at slower time strata.
They do seem to be getting remarkable resolutions. -
Unclear Engineer The description of the effects on resolution from the distribution of the radio telescopes on Earth got me thinking about what might be achieved with radio telescopes added on the Moon, or even the Moon plus L2 and or L1. Add in L4 and L5, and we should be able to "see" some mighty fine details.Reply
That is the kind of science I hope we can do when Starship is able to put up much more massive payloads reliably and less expensively. -
Torbjorn Larsson More accurate estimates of the black holes' spin rates and angle to us are essential for a new Sgr A* merger model:Reply
Inspired by the merger between the Milky Way and the Gaia-Enceladus progenitor, which had a 4:1 mass ratio as inferred from Gaia data, we have discovered that a 4:1 major merger of a SMBH with a binary angular momentum inclination angle of 145–180° with respect to the line of sight can successfully replicate the measured spin properties of Sgr A*. This possible merger event in our Galaxy’s history provides potential observational support for the theory of hierarchical black hole mergers in the formation and growth of SMBHs. The inferred merger rate ... consistent with theoretical predictions ...
-
Torbjorn Larsson
The geometry of spacetime is what explains the light bending of Sun, as opposed to the old newtonian gravitation approximation you refer to which predicts only half of the observed bending. This is, or should be, well know historical facts:Questioner said:Light follows the geometry of space-time exclusively.
The gravitational effect can not be involved because light/EM has no resting mass to favor/linger at slower time strata.
Henry Cavendish in 1784 (in an unpublished manuscript) and Johann Georg von Soldner in 1801 (published in 1804) had pointed out that Newtonian gravity predicts that starlight will bend around a massive object. The same value as Soldner's was calculated by Einstein in 1911 based on the equivalence principle alone. However, Einstein noted in 1915 in the process of completing general relativity, that his 1911 result (and thus Soldner's 1801 result) is only half of the correct value. Einstein became the first to calculate the correct value for light bending: 1.75 arcseconds for light that grazes the Sun.
https://en.wikipedia.org/wiki/Tests_of_general_relativity
The first observation of light deflection was performed by noting the change in position of stars as they passed near the Sun on the celestial sphere. The observations were performed by Arthur Eddington and his collaborators (see Eddington experiment) during the total solar eclipse of May 29, 1919, when the stars near the Sun (at that time in the constellation Taurus) could be observed. Observations were made simultaneously in the cities of Sobral, Ceará, Brazil and in São Tomé and Príncipe on the west coast of Africa. The result was considered spectacular news and made the front page of most major newspapers. It made Einstein and his theory of general relativity world-famous.