What happens inside neutron stars, the universe's densest known objects?
We don't really know, but probably some truly bizarre physics.
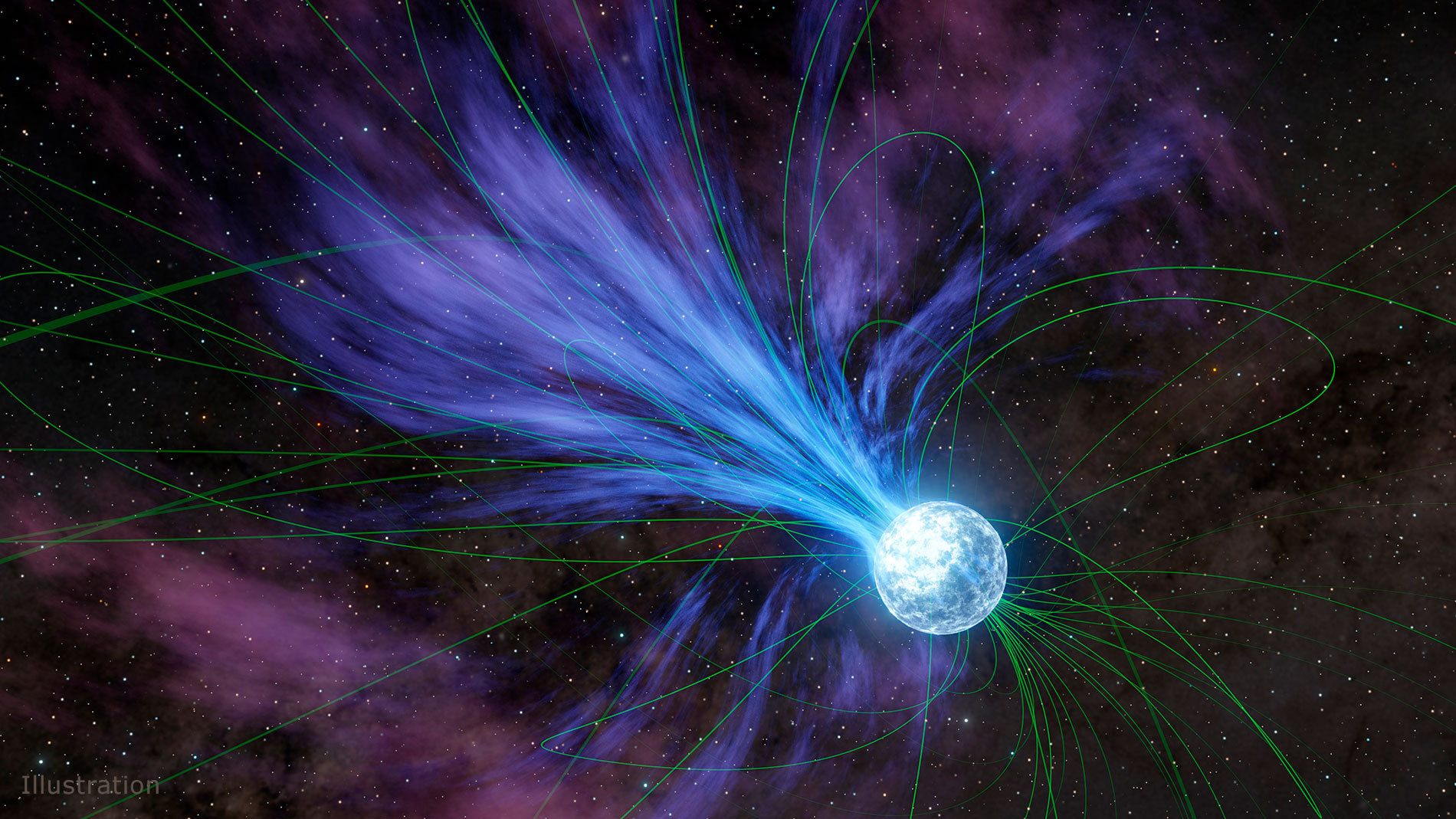
Neutron stars are some of the weirdest cosmic objects, and the greatest mysteries lie deep in their hearts.
Neutron stars are the leftover cores of exploded stars and the densest known material in the universe. A typical neutron star has a mass a few times the mass of the sun, compressed into a region only a dozen kilometers across. In the outermost layers of neutron stars, the density is billions of times greater than the density of a diamond. In the cores of neutron stars, the crushing pressures can squeeze apart atomic nuclei, and maybe even protons and neutrons themselves.
Neutron stars' surface gravities are so intense that the largest "mountains" are only a few millimeters tall. And those surfaces are already alien, consisting of a crust of heavy atomic nuclei squeezed into a crystalline lattice with electrons free to swim among them.
Deeper down, the enormous gravity allows for exotic, rare isotopes to exist in abundance. Usually, you can only cram so many neutrons into a nucleus before it just falls apart, due to the repulsive effects of the strong nuclear force resisting cohesion. But the gravity of the neutron star keeps everything bound together. Down to a depth of roughly a kilometer, atomic nuclei can hold hundreds of neutrons at a time.
But even that has a limit. At a little over half a mile (1 km) in depth, even those impossibly sized nuclei break down. This is the "drip line," where neutrons begin to leak out of nuclei. Normally, any free neutrons decay in roughly 15 minutes. But the intense confines of the neutron star's interior keep the neutrons stable and free-flowing.
After only about a mile (2 kilometers) deep into the neutron star, matter takes on perhaps its strangest form yet: the "nuclear pasta." In this region, where the crust transitions to the core, titanic forces — gravity, the strong nuclear force and electric repulsion — compete for dominance. This leads to odd, lumpy nuclei with exaggerated shapes, known as gnocchi.
Below that, the individual lumps squeeze together into long (relatively speaking; everything here is still microscopic) tubes, known as spaghetti. Then, the spaghetti fuse together to form lasagne, which then merge into a single uniform mass. But that mass has defects and holes in it — the "antispaghetti" and "antignocchi."
Get the Space.com Newsletter
Breaking space news, the latest updates on rocket launches, skywatching events and more!
All told, the nuclear pasta region is only about 330 feet (100 meters) thick, but it weighs more than 3,000 Earths. That's a lot of pasta.
Below that, at a depth of about a mile, the nuclei simply break down, as they're unable to maintain their structures in the crushing environment. Here, neutrons, protons and some electrons roam freely. And what truly happens in the core region of a neutron star is a matter of much debate, because the physics here is so far beyond our current understanding.
We strongly suspect that the outer region of the core is a superfluid, where the neutrons are free to move with zero viscosity and zero friction. The remaining protons at this depth are now also a superconductor, with no electrical resistivity. At these depths, the density is comparable to an atomic nucleus, with the protons and neutrons squeezed together as tightly as they can be. This region of a neutron star is, for all intents and purposes, a single macroscopic atomic nucleus, bound together not by the strong nuclear force but by sheer gravitational might.
The neutrons in this region do a large part of the work in supporting the star against further gravitational collapse. One way is through "degeneracy pressure" — they are squeezed so tightly together that they buzz with incredible, near-light-speed velocities, which creates pressure. Alongside that, the strong nuclear force is repulsive between neutrons, which keeps them from squeezing together even more.
In the deepest regions of the core, however, we simply have no idea. The densities in the innermost core are higher than in an atomic nucleus. We have no hope of replicating or recreating those conditions in the laboratory, so we only have hazy mathematical models to guide us. In some models, the neutrons maintain their superfluid state.
In other models, different forms of matter — like hyperons, deltas and boson condensates — may arise. This is possible because neutrons and protons are made of even smaller particles, called quarks. In these conditions, the quarks may arrange and combine themselves differently in situations that would be instantly unstable in any other environment. But here, they may be perfectly fine.
In other models, all protons and neutrons — and even their more exotic cousins — break down completely, forming a soup of quarks and gluons, the carriers of the strong nuclear force.
But all of this is pure speculation. The nearest neutron star is hundreds of light-years away, and even if we could crack it open, the special conditions that create these kinds of exotic conditions would break down. So, for now, the only way to peer inside neutron stars is with math and a heavy dose of guesswork.
Join our Space Forums to keep talking space on the latest missions, night sky and more! And if you have a news tip, correction or comment, let us know at: community@space.com.
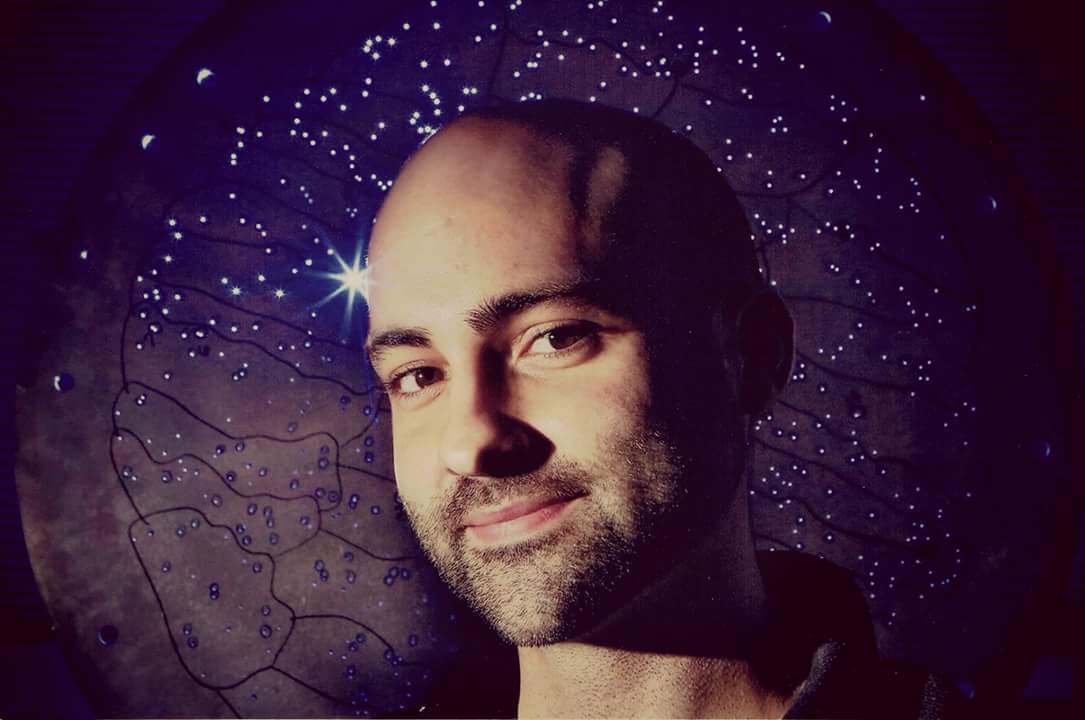
Paul M. Sutter is an astrophysicist at SUNY Stony Brook and the Flatiron Institute in New York City. Paul received his PhD in Physics from the University of Illinois at Urbana-Champaign in 2011, and spent three years at the Paris Institute of Astrophysics, followed by a research fellowship in Trieste, Italy, His research focuses on many diverse topics, from the emptiest regions of the universe to the earliest moments of the Big Bang to the hunt for the first stars. As an "Agent to the Stars," Paul has passionately engaged the public in science outreach for several years. He is the host of the popular "Ask a Spaceman!" podcast, author of "Your Place in the Universe" and "How to Die in Space" and he frequently appears on TV — including on The Weather Channel, for which he serves as Official Space Specialist.
You must confirm your public display name before commenting
Please logout and then login again, you will then be prompted to enter your display name.